Application of Magnetic Particles in Organelle Separation
In bioanalytical chemistry, it is very important to isolate organelles to analyze their content or function, which is the basis of many biomedical analyses. Typically, following the preparation of a cell homogenate using mechanical homogenization, there will be a mixture of various organelle types. Then, by using immunoaffinity separation or centrifugation to fractionate the organelles, we can obtain enriched target organelle fractions. During this process, low levels of organelle contamination, high sensitivity of relevant analytical techniques, compatibility with low sample volumes, and the ability to obtain subcomponents of specific organelle types are required. Scientists have developed many better enrichment techniques to meet these needs in bioanalytical chemistry. Here, we focus on the application of magnetic particles to the immunoaffinity separation of organelles.
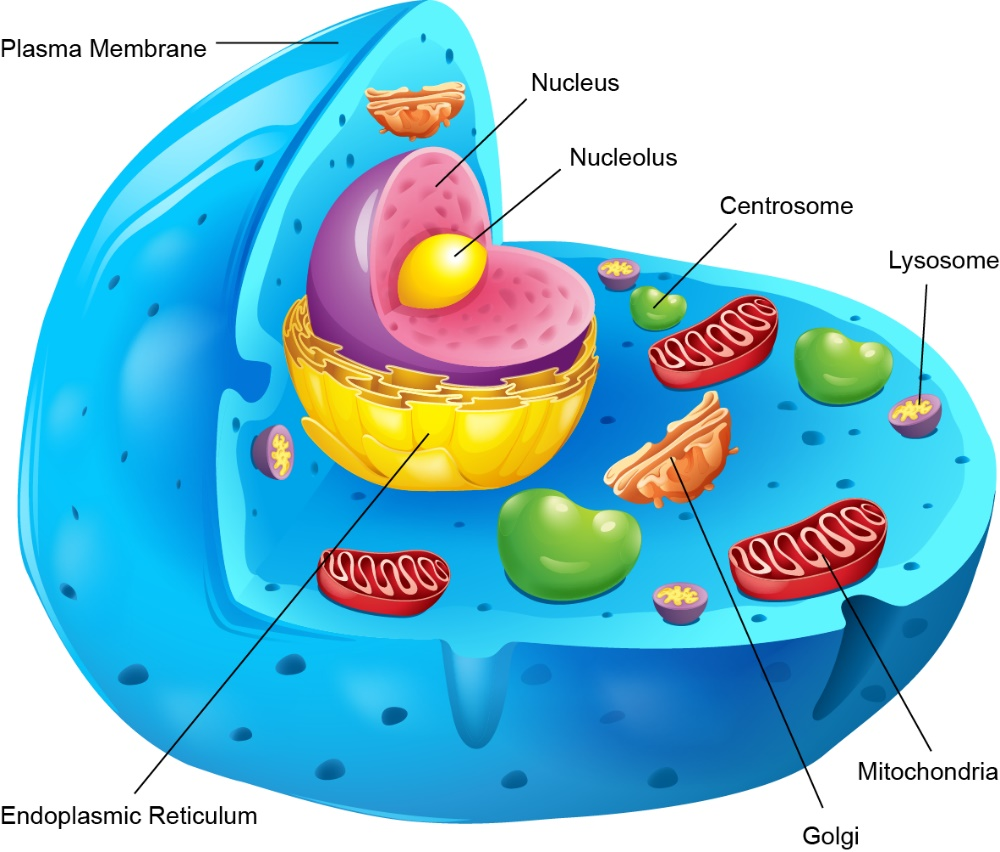
Figure 1: Schematic diagram of organelles inside the cell
Immunoaffinity isolation of organelles, which is based on the interaction between antibodies and antigens or proteins found on the surface of the organelle of interest, is also a limiting factor. However, this landscape is beginning to change with the increasing number of suitable antibodies and the ability to assess the functional status of immunopurified organelles. There are many reports on the isolation of organelles using antibody-coupled magnetic particles, including the isolation of synaptic vesicles, exosomes, chloroplasts, mitochondria, peroxisomes, and lysosomes.
Synaptic Vesicle
Synaptic vesicles are probably the most abundant vesicular organelles, and their function and molecular composition have been well studied due to their high degree of purification. They are spherical at the electron microscope level, with a characteristic cross-sectional diameter of approximately 40 nm ± 4% to 9%. Synaptic vesicles can be purified by differential centrifugation, density-based precipitation, and size fractionation, and today they can also be purified by magnetic particles conjugated to various antibodies that recognize synaptic vesicle proteins. Burré et al released synaptic vesicles from rat brains by hypotonic shock, followed by purification using sucrose density gradient centrifugation. They also purified specific gradient fractions by immune separation using four different types of magnetic particles. The results showed that M-280 goat anti-mouse beads were the most efficient group, providing pure synaptic vesicle samples with low non-specific binding for subsequent experiments.
Liquid exosomes are a subpopulation of extracellular vesicles with a diameter of approximately 30 to 100 nm. They are composed of lipids, carbohydrates, proteins, and nucleic acids and can serve as a means of intercellular communication, being released from the cell of origin to initiate different functions on the target cell. Using targeted exosome-specific markers, specific exosomes can be captured with increasing purity. This strategy is also ideal for removing co-enriched exosomes and isolating exosome subpopulations from media supplements. Considering the compatibility with subsequent analysis, the bead-based method is general for specific capture. While latex beads have been used for specific capture, the protocol involves many centrifugation steps and is challenging to reproducible. Therefore, magnetic separation of magnetic particles is a better alternative to latex beads for exosome capture, which can isolate more uniform populations of vesicles in terms of size, morphology, and protein content. Clayton et al. developed a rapid and versatile technique for exosome isolation. This method uses anti-MHC class II antibodies coated onto larger solid surfaces (4.5 μm magnetic particles) for downstream analysis.
Chloroplast
The chloroplast structure of different cell types is different. However, isolating chloroplasts from specific cells is often difficult, so we do not know much about the relationship between different chloroplast structures and functions. Truenit et al. developed a method to label the surface of chloroplasts from individual cell types with an exogenous yellow fluorescent protein and then isolate these chloroplasts by immunogenic methods. After homogenizing whole leaves, both labeled and unlabeled chloroplasts are released, and labeled chloroplasts can be isolated by magnetic particles coated with an antibody to GFP. They found that the abundance of various chloroplast transcripts differed between Arabidopsis spongy mesophyll cells, vascular cells, and epidermal cells. Moreover, this method is based on genetic logic and thus can also be used to isolate organelles or subcellular compartments from other transformable organisms.
Mitochondria
Mitochondria play an important role in maintaining the life and death of cells by regulating key functions of cells. Thus, mitochondrial dysfunction can lead to a variety of human diseases and may be associated with cancer, cardiovascular disease, neurodegenerative diseases, etc. Developing techniques to access mitochondrial targets for the treatment of diseases associated with mitochondrial dysfunction is of increasing interest and urgency. Banik et al. reported a mitochondria-targeting magnetic nanoparticle (Mito-magneto), which can efficiently isolate mitochondria without the need for centrifugation and immunoprecipitation (Fig. 1). Mito-magneto is a Fe3O4 magnetite-based magnetic nanoparticle whose surface is functionalized with triphenylphosphine (TPP) cations. This magnetic nanoparticle can separate mitochondria under the influence of a magnetic field, and the obtained mitochondria are intact, pure, and respiratory. In addition, it eliminates artifacts commonly associated with the centrifugation of nanoparticle-loaded mitochondria.
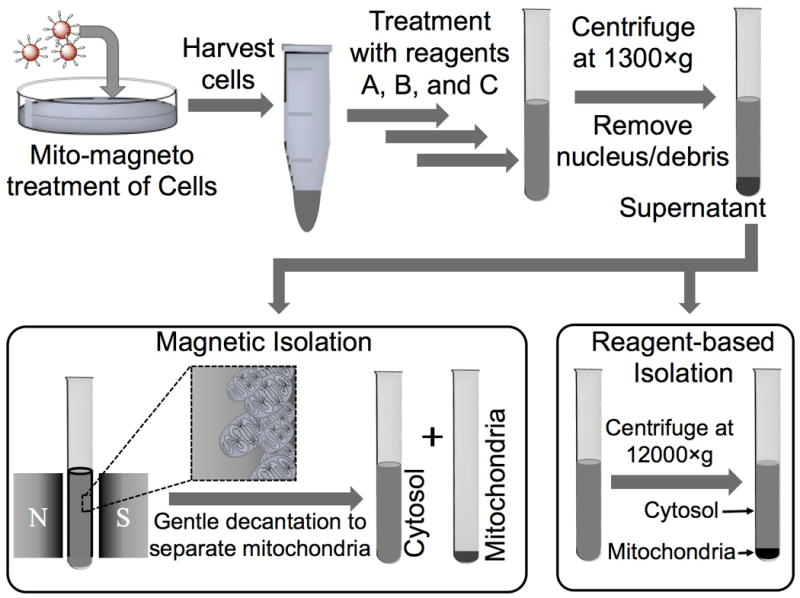
Figure 2: Schematic diagram of the mitochondrial isolation procedure using magnetic and reagent-based
Peroxisome
Peroxisomes that generate reactive oxygen species may be involved in the biotransformation of innate biomolecules and xenobiotics. To avoid confusion with biotransformations occurring in other co-isolated organelles, it is important to isolate functional peroxisomes with fewer contaminants to study biotransformations occurring in these organelles. Wang et al immobilized an antibody against a 70 kDa peroxisome membrane protein on siliconized magnetic iron oxide particles coated with protein a, and then they magnetically captured peroxisomes from L6 rat myoblast homogenate , followed by washing and analysis of subcellular composition by enzymatic assays. The results showed that peroxisomes were more pure and retained their biological activity.
Lysosome
Lysosomes are membrane-bound organelles with digestive enzymes. They are involved in a variety of cellular processes, including breaking down redundant or damaged cell parts, destroying invading viruses and bacteria, and apoptosis. Lysosomes have V-
Expression of ATPase. Based on this, Nylandsted et al. used an anti-V-ATPase antibody to enrich lysosomes during the immunopurification procedure. Organelles retained within the magnetic column are acidic in nature. The results of the proteomic analysis showed that the subcellular localization of the enriched proteins mainly came from lysosomes and some endosomes. Furthermore, only one mitochondria-associated protein was found, and no Golgi, plasma membrane, and endoplasmic reticulum integral membrane proteins were detected, suggesting that the method is sufficient for purifying lysosomes.
References
1.Šafařík, I., & Šafaříková, M. (2002). Magnetic nanoparticles and biosciences. In nanostructured materials (pp. 1-23). Springer, Vienna. https://link.springer.com/chapter/10.1007/978-3-7091-6740-3_1
2.Burré, J., Zimmermann, H., & Volknandt, W. (2007). Immunoisolation and subfractionation of synaptic vesicle proteins. Analytical biochemistry, 362(2), 172-181. https://doi.org/10.1016/j.ab.2006.12.045
3. Truernit, E., & Hibberd, J. M. (2007). Immunogenic tagging of chloroplasts allows their isolation from defined cell types. The Plant Journal, 50(5), 926-932. https://doi.org/10.1111/j.1365-313X.2007.03113.x
4. Wang, Y., Taylor, T. H., & Arriaga, E. A. (2012). Analysis of the bioactivity of magnetically immunoisolated peroxisomes. Analytical and bioanalytical chemistry, 402(1), 41-49. https://link.springer.com/article/10.1007/s00216-011-5476-3
5.Satori, C. P., Kostal, V., & Arriaga, E. A. (2012). Review recent advances in the analysis of isolated organelles. Analytica chimica acta, 753, 8-18. https://doi.org/10.1016/j.aca.2012.09.041
6.Banik, B., & Dhar, S.(2018). Centrifugation‐Free Magnetic Isolation of Functional Mitochondria Using Paramagnetic Iron Oxide Nanoparticles. Current protocols in cell biology, 76(1), 25-4. https://doi.org/10.1002/cpcb.26
7.Oksvold, M. P., Neurauter, A., & Pedersen, K. W.(2015). Magnetic bead-based isolation of exosomes. In RNA Interference(pp. 465-481). Humana Press, New York, NY. https://link.springer.com/protocol/10.1007/978-1-4939-1538-5_27
8.Tchikov, V., Fritsch, J., Kabelitz, D., & Schütze, S. (2010). Immunomagnetic isolation of subcellular compartments. In Methods in Microbiology (Vol. 37, pp. 21-33). Academic Press. https://doi.org/10.1016/S0580-9517(10)37002-4
Aladdin:https://www.aladdinsci.com
List of related products