Nanoparticle-Based Nucleic Acid Delivery
Nucleic acid therapy, as a prominent, novel and promising therapeutic approach, has potential in the treatment of many genetic diseases such as diabetes. Nucleic acid therapy consists of two parts: 1) a therapeutic nucleic acid containing plasmid DNA (pDNA) and small RNA; 2) a vector that delivers the nucleic acid to the target. Therapeutic nucleic acids include pDNA, antisense oligonucleotides (AON), and RNA molecules. These molecules and RNA interference (siRNA, shRNA, miRNA) are generally used in nucleic acid therapy, among which siRNA and pDNA are important nucleic acid types for gene therapy, mainly as potential therapeutic agents for silencing the expression of defective genes. Vectors are divided into viral vectors and non-viral vectors. Viral vectors include retroviruses, adenoviruses, and lentiviruses, which are more efficient than nonviral vectors in many cell lines. As for viral vectors, the packaging and unpackaging of nucleic acids inside/outside the capsid is controlled, and the ability of viruses enables them to overcome many extracellular and intracellular delivery barriers or defense mechanisms of target cells. However, viral vectors also have some disadvantages, such as severe off-target immunogenicity, inflammatory response, and toxicity. Nonviral vectors include cationic lipids, polymers, and peptides that mimic key features of viral vectors to compress and deliver nucleic acids in a similar manner. Due to the artificial design, non-viral vectors are usually not immediately recognized by the immune system, which makes them much safer than viral vectors. Moreover, the chemical structure of non-viral vectors is controllable, making it easier to synthesize on a large scale and commercially. The challenges faced by non-viral vectors in designing suitable delivery methods are as follows:
• Improving the efficiency of passive targeting
• Increasing intracellular bioavailability
• Inducing immune response
• low the nuclear uptake
• Extracellular and Intracellular Barriers in Nucleic Acid Delivery (Figure 1)
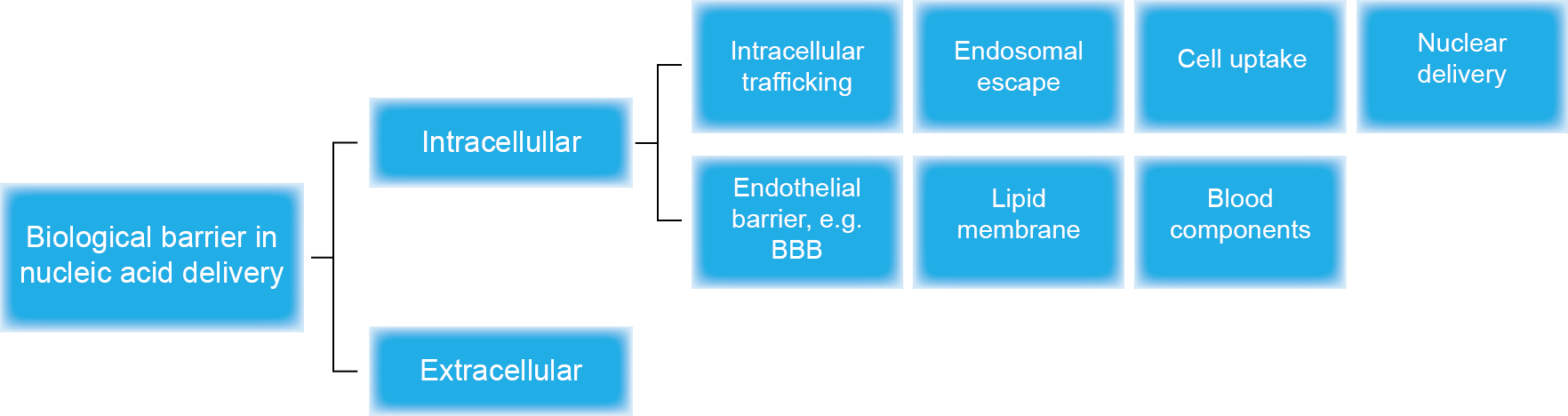
Figure 1: Different types of biological barriers in nucleic acid delivery; BBB: blood-brain barrier
Nanoparticles have the advantages of good biocompatibility, low immunogenicity, selective targeting, short circulation time, effective penetration of various barriers, and no serious side effects during self-release, making them a promising nucleic acid delivery vehicle. Nanoparticles can wrap and trap many biomolecules or drugs onto their surface by chemical coupling or adsorption, among which lipids, gold, silica nanoparticles, and quantum dots have been developed as new nanocarriers for the nucleic acid is delivered to the targeted site.
Lipid-Based Nanoparticles
Lipid-based nanoparticles have been developed for nucleic acid delivery. The cationic liposome is the key to this system because it condenses the DNA into cationic nanoparticles when the two components are mixed together. This cationic lipid/DNA complex prevents enzymatic degradation of DNA and transports DNA into cells by interacting with negatively charged cell membranes. However, these cation-based delivery systems face several disadvantages, such as low gene transfer efficiency, short circulation time, and toxicity associated with inflammation and complement activation. Therefore, scientists developed a pegylated cationic liposome bilayer to encapsulate nucleic acid material by various methods. The system has a double shell around the nucleic acid, a smaller particle size (less than 100 nm), and better in vivo stability. Hope et al. developed dialkylamine iL nanoparticles (iLNPs, using amino lipids as core polymers) to enhance siRNA delivery at the target site. They found that siRNA entry into hepatocytes was accelerated through this delivery system.
Gold Nanoparticles
Gold nanoparticles (AuNPs) can be stabilized by various stabilizers such as polymers, ligands, biomolecules, dendrimers, and surfactants. However, these stabilizers not only affect relative instability and aggregation, but also cellular uptake and toxicity. Therefore, it is very important to engineer the surface of AuNPs so that they can be applied to gene delivery and as nucleic acid carriers. In 2001, McIntosh et al. reported that AuNPs were functionalized with cationic quaternary ammonium compounds and then bound to pDNA by electrostatic interaction. This unique composition protects pDNA from enzymatic degradation and also regulates transcription by RNA polymerase.
Silicon Nanoparticles
Inhibiting gene expression at the mRNA and protein levels through Watson-Crick base pairing. These molecules are promising therapeutics for diseases with an underlying genetic component, such as viral infections and cancer. However, they also suffer from issues such as poor intracellular uptake and stability, resulting in limited therapeutic use. developed poly-l-lysine-modified silica nanoparticles (PMS-NPs) to bind and protect AON. They found that PMS-NPs exhibited significantly lower cytotoxicity in human nasopharyngeal carcinoma cells and HeLa cells.
Quantum Dot
Quantum dots (QDs) have unique optical properties that make them ideal vectors for size- and shape-tunable imaging. They also feature narrow symmetric bands of emission over a broad excitation range, stability against photobleaching, and availability to be functionalized over large surface areas. In addition, quantum dots play an important role in improving the efficiency and tracking of siRNA in target cells.
The distribution of siRNAs in cells in vivo or in vitro shows great potential. Wu et al. synthesized a functional CdSe/ZnS quantum dot complex QD-SMCC/siSOX 9 . Polyethylene glycol-coated quantum dots were activated using sulfosuccinimidyl-4-(n-maleimidomethyl)cyclohexane-1-carboxylate (sulfo-SMCC ). The QD-SMCC/si SOX 9 is used as a gene carrier of siRNA to explore the SOX9 Effects of RNA interference on the chondrogenic differentiation of mesenchymal stem cells (MSCs). The results showed that QD-SMCC could effectively bind and deliver siRNA into MSCs. The transfection efficiency of QD-SMCC was 68.6%, which was better than 56.4% of PEI. In addition, the ZnS shell and PEG coating also greatly reduced the cytotoxicity of QD-SMCCs.
References
1. Xiao, Y., Shi, K., Qu, Y., Chu, B., & Qian, Z. (2019). Engineering nanoparticles for targeted delivery of nucleic acid therapeutics in tumors. Molecular Therapy-Methods & Clinical Development, 12, 1-18.https://doi.org/10.1016/j.omtm.2018.09.002
2. Sharma, A. K., Gupta, L., & Gupta, U. (2017). Nanoparticles as nucleic acid delivery vectors. In Advances in Nanomedicine for the Delivery of Therapeutic Nucleic Acids (pp. 13-42). Woodhead Publishing. https://doi.org/10.1016/B978-0-08-100557-6.00002-X
3.He, Z. Y., Jin, Z. H., Zhan, M., Qin, Z., Li, Z., & Xu, T. (2017). Advances in quantum dot-mediated siRNA delivery. Chinese Chemical Letters, 28(9), 1851-1856. https://doi.org/10.1016/j.cclet.2017.07.012
4. Mei, Y., Wang, R., Jiang, W., Bo, Y., Zhang, T., Yu, J., ... & Ma, W. (2019). Recent progress in nanomaterials for nucleic acid delivery in cancer immunotherapy. Biomaterials science. https://doi.org/10.1039/C9BM00214F
5. Mukalel, A. J., Riley, R. S., Zhang, R., & Mitchell, M. J. (2019). Nanoparticles for nucleic acid delivery: Applications in cancer immunotherapy. Cancer Letters, 458, 102-112. https://doi.org/10.1016/j.canlet.2019.04.040
6. Hope, M. J. (2014). Enhancing Si-RNA delivery by employing lipid nanoparticles. Therapeutic delivery, 5(6), 663-673. https://doi.org/10.4155/tde.14.37
7.McIntosh, C. M., Esposito, E. A., Boal, A. K., Simard, J. M., Martin, C. T., & Rotello, V. M. (2001). Inhibition of DNA transcription using cationic mixed monolayer-protected gold clusters. Journal of the American Chemical Society, 123(31), 7626-7629. https://doi.org/10.1021/ja015556g
8. Zhu, S. G., Xiang, J. J., Li, X. L., Shen, S. R., Lu, H. B., Zhou, J., ... & Tang, K. (2004). Poly (l‐lysine)‐modified silica nanoparticles for the delivery of antisense oligonucleotides. Biotechnology and applied biochemistry, 39(2), 179-187. https://doi.org/10.1042/BA20030077
9. Wu, Y., Zhou, B., Xu, F., Wang, X., Liu, G., Zheng, L., ... & Zhang, X. (2016). Functional quantum dot-Si-RNA nanoplexes to regulate chondrogenic differentiation of mesenchymal stem cells. Acta biomaterialia, 46, 165-176. https://europepmc.org/article/med/27615736