PEG-Azide-Alkyne—Bioorthogonal and Click Chemistry
Introduction of Bioorthogonal Chemistry
• pH: The reaction must occur at the temperatures and pH of physiological environments.
• Efficient: The reaction must provide products selectively and in high yields and must not be affected by water or endogenous nucleophiles, electrophiles, reductants, or oxidants found in complex biological environments.
• Fast: the reaction must be fast, even at low concentrations, and must form stable reaction products.
• Specific: The reaction should involve functional groups not naturally present in biological systems.
Strengths and Weaknesses of Bioorthogonal Reactions
Bioorthogonal reactions | Advantages | Disadvantages |
Staudinger ligation | Azides and phosphines are biocompatible, stable amide linkages. | Slow reactions, phosphines prone to oxidation. |
CuAAC (azide + alkyne) | Fast reactions, k~10-100(M-1S-1) with 20 μM Cu(I). Good regioselectivity. | Despite some ligands such as THPTA to stabilize copper catalysts, copper toxicity remains a concern. |
SPAAC (azide + DBCO) | No use of copper catalysts k~1-60 (M-1S-1) | 1. Reactions are slower than CuAAC, bulky cyclooctynes are difficult to incorporate into biomolecules. 2. Preferred solvent: ethanol (10-40%) or DMSO (up to 60%)/ PBS buffer. 3. pH < 5.5 run slow down because of low stability DBCO. 4. Thiol, sodium azide reacts with DBCO. |
IEDDA (TCO + Tz) | Very fast reactions, k~1-106 (M-1S-1) | TCO has lower stability in aqueous environments. |
Introduction of Azide-Alkyne Click Chemistry
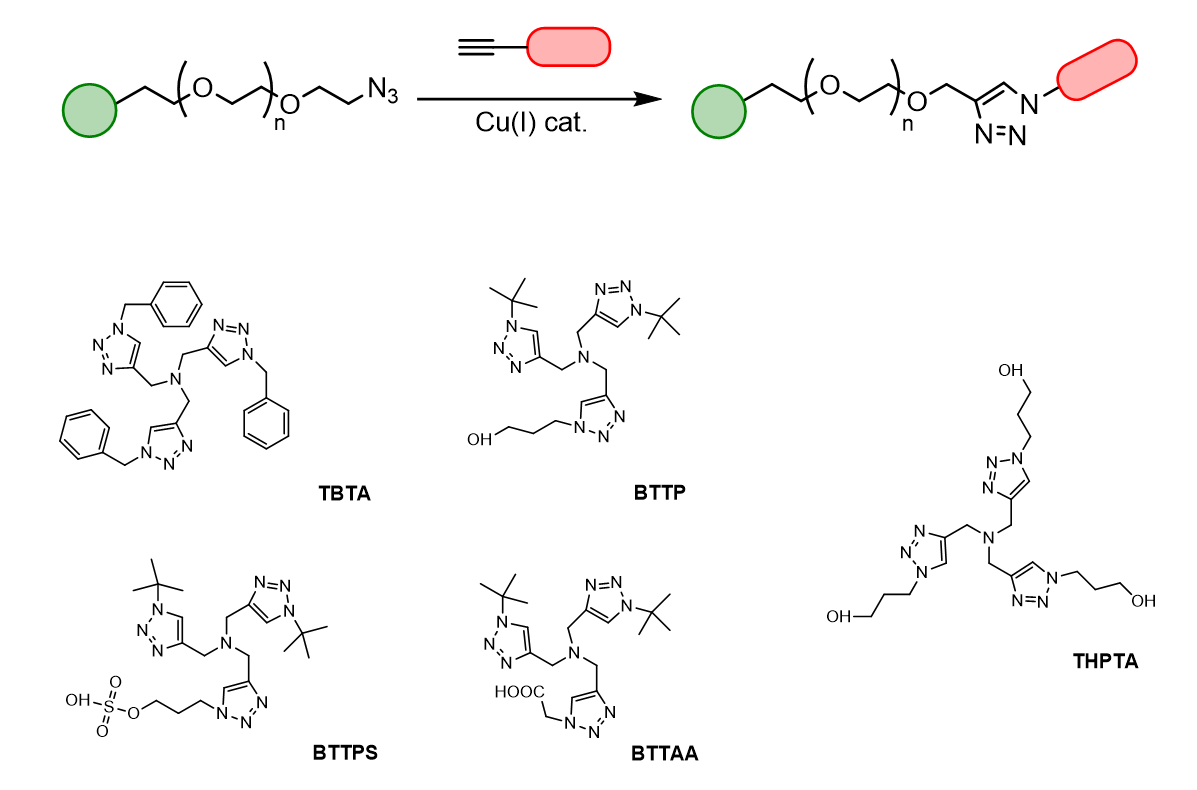
A modification of click chemistry uses the reduction of CuSO4 with sodium ascorbate and Cu(I) -stabilizing ligand tris (benzyl triazolyl methyl) amine (TBTA) to prepare Cu(I) in situ. This leads to a more reliable click reaction by avoiding catalyzing Cu(I) oxidation by dissolved oxygen. In a typical reaction, copper sulfate is precomplexed with TBTA. This composite catalyst was mixed with alkyne and azide, and then sodium ascorbate was added to initiate the click reaction.
TBTA covers some practical applications of click chemistry, except for aqueous conjugation reactions. The water-soluble tris (3-hydroxypropyl triazolyl methyl) amine (THPTA) click ligand further simplifies click chemistry by allowing the entire reaction to run in water, providing biocompatibility for the click reaction.4 The THPTA ligands bind Cu(I), blocking Cu(I) bioavailability and ameliorating potential toxic effects while maintaining its catalytic efficiency. THPTA ligands efficiently label viable cells while maintaining cell viability.5
In our research, we found THPTA to be an efficient ligand for click chemistry, partially in a complete aqueous reaction. The labelling was completed for 15 to 30 min at room temperature. The ligand CuSO4 complex did not exhibit loss of activity when frozen for at least one month.
Example protocols
Labeling of oligonucleotides and DNA1. Prepare the following stock solutions
• 200 mM THPTA ligand in water
• 100 mM CuSO4 in water
• alkyne labeled oligo in water
• 100 mM sodium ascorbate in water
• 10 mM azide in DMSO/t-BuOH or water
2. Incubate CuSO4 with THPTA ligand in a 1:2 ratio several minutes before the reaction. This solution is stable for several weeks when frozen.
3. To the oligo/DNA solution, add an excess of azide (4-50 eq).
4. Add 25 equivalents of THPTA/CuSO4.
5. Add 40 equivalents of sodium ascorbate.
6. Let the reaction stand at room temperature for 30-60 minutes.
7. Ethanol-precipitate the oligo or purify.
Labeling of cell lysate
• 100 mM THPTA ligand in aqueous buffer or water
• 20 mM CuSO4 in water
• 300 mM sodium ascorbate in water
• 2.5 mM alkyne or azide labeling reagent in water or DMSO
2. For each azide or alkyne- modified protein lysate sample, add the following to a 1.5 mL microfuge tube, then vortex briefly to mix.
• 50 µL protein lysate (1-5 mg/mL) in protein extraction buffer
• 90 µL PBS buffer
• 20 µL of 2.5 mM corresponding azide (or alkyne) detection reagent in DMSO or water
3. Add 10 µL of 100 mM THPTA solution, vortex briefly to mix.
4. Add 10 µL of 20 mM CuSO4 solution, vortex briefly to mix.
5. Add 10 µL of 300 mM sodium ascorbate solution to initiate click reaction, vortex briefly to mix.
6. Protect reaction from light and allow click reaction to incubate for 30 minutes at room temperature.
7. Proteins in lysate are now click labeled and ready for downstream processing and/or analysis.
Labeling live cells by copper-catalyzed alkyne-azide click chemistry
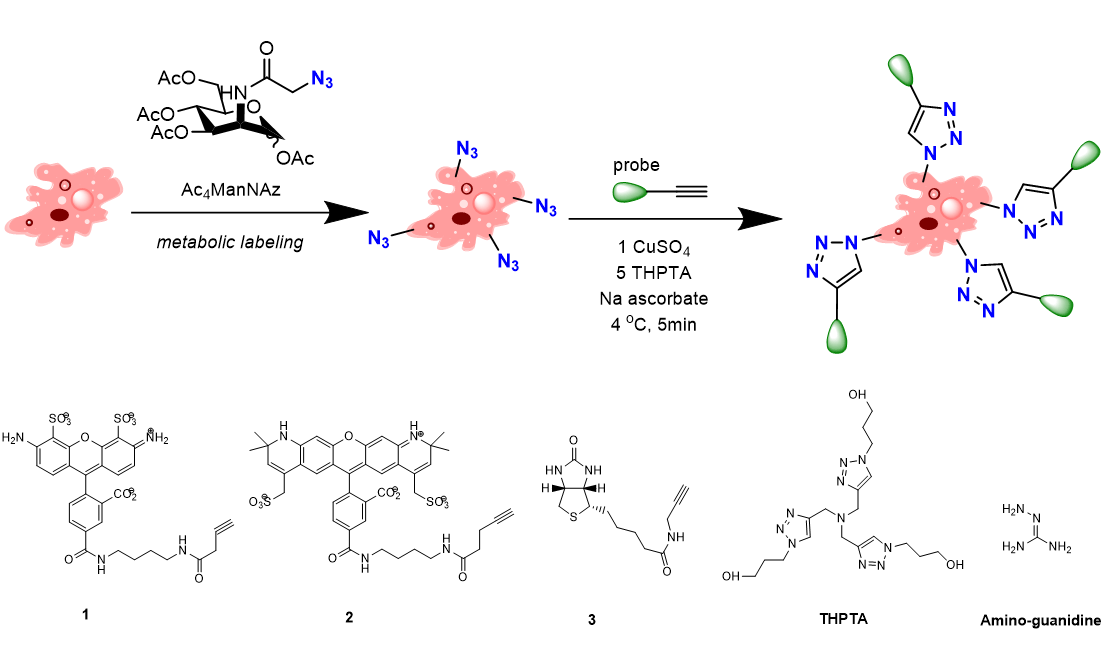
Figure1. (Top) Cell labeling steps.
Cell-surface labeling of azido glycans on HeLa and CHO cells and imaging by confocal microscopy
Cells were seeded at 1 × 105 cells/mL on glass-bottom Petri dishes (35 mm) and grown overnight at 37 °C and 5% CO2 in growth medium (MEM medium containing 10% fetal calf serum, 1% glutamine, and 1% penstrep) with or without 50 μM Ac4ManNAz for 2 days. The medium was gently aspirated, and the cells were washed two times with 1 mL of DPBS. In an eppendorf tube, CuSO4 and THPTA in a 1:5 molar ratio was added to DPBS at 4 °C containing dye-alkynes 1 or 2 (final conc. 25 μM) and aminoguanidine (final conc. 1 mM). A freshly prepared stock solution of sodium ascorbate (100 mM) was added to establish a final ascorbate concentration of 2.5 mM. This reaction mixture was incubated on ice for 10 min at 4 °C before adding to the cells. After incubation at 4 °C for 1or 5 min, the cells were washed and fixed with a mixture of 3% paraformaldehyde, 0.3% glutaraldehyde, and 1 mM MgCl2 in DBPS for 10 min at room temperature. Cell nuclei were stained by adding 4′,6-diamidino-2-phenylindole (DAPI). Between each step, the slides were rinsed three times with DPBS. Slides were mounted using Vecta Shield mounting medium (Vector Laboratories, Burlingame, CA). Sections were imaged using a Bio-Rad 2100 confocal microscope with a 60× oil objective. Data were analyzed and images were created using ImageJ (http://rsbweb.nih.gov/ij/). For dual labeling studies, the cells were washed twice with 1 mL of growth medium after the labeling reaction and returned to medium containing 50 μM Ac4ManNAz for another 20 h. Optimized conditions for cellsurface labeling were 25 μM alkyne-488, CuSO4 (50 μM), THPTA (250 μM), aminoguanidine (1 mM), and sodium ascorbate (2.5 mM) for 1 to 5 min in medium at 4 °C.
References
2. Analysis and Optimization of Copper-Catalyzed Azide–Alkyne Cycloaddition for Bioconjugation; V. Hong, S. I. Presolski, C. Ma, M. G. Finn, Angew. Chem. Int. Ed. 2009, 48, 9879. https://onlinelibrary.wiley.com/doi/10.1002/ange.200905087
3. Stabilization of Virus-like Particles with Poly(2-oxazoline)s; F. Manzenrieder, R. Luxenhofer, M. Retzlaff, R. Jordan, M. G. Finn, Angew. Chem. Int. Ed. 2011, 50, 2601. https://onlinelibrary.wiley.com/doi/10.1002/ange.201006134
4. Synthesis of Cyclic Peptide Disulfide–PHPMA Conjugates via Sequential Active Ester Aminolysis and CuAAC Coupling; K. A. Günay, H. Klok, Polym. Chem. 2016, 7, 970. https://pubs.rsc.org/en/content/articlelanding/2016/py/c5py01817j
5. Cellular Consequences of Copper Complexes used to Catalyze Bioorthogonal Click Reactions; D. C. Kennedy, C. S. McKay, M. C. B. Legault, D. C. Danielson, J. A. Blake, A. F. Pegoraro, A. Stolow, Z. Mester, J. P. Pezacki, J. Am. Chem. Soc. 2011, 133, 17993. https://pubs.acs.org/doi/10.1021/ja2083027